Science Mapping Analysis of Density Functional Theory (DFT) for Material Design: A Review
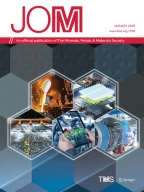
This review explores the applications of density functional theory (DFT) in materials design and how DFT has revolutionized materials discovery for various fields, including drug delivery, energy storage, and spacecraft coatings. The science mapping analysis identifies research trends, and the dominant countries leading the development of new materials for future advancements are reviewed. Fluorescent and localized materials for cancer treatment, thin films for energy storage, catalysts, and materials resistant to spacecraft, efficient energy storage, powerful catalysts, and more reliable spacecraft coatings for future exploration and research are discussed. We found that the strongest research growth was mainly demonstrated by the United States due to its research capabilities, funding, active collaboration, and emphasis on innovation in the design of new materials for various applications.
This is a preview of subscription content, log in via an institution to check access.
Access this article
Subscribe and save
Springer+ Basic
€32.70 /Month
- Get 10 units per month
- Download Article/Chapter or eBook
- 1 Unit = 1 Article or 1 Chapter
- Cancel anytime
Buy Now
Price includes VAT (France)
Instant access to the full article PDF.
Rent this article via DeepDyve
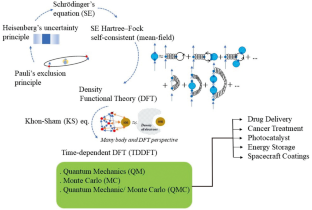
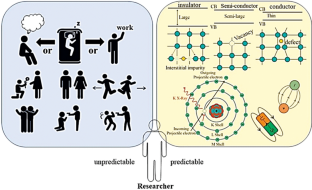
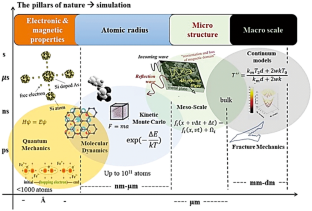
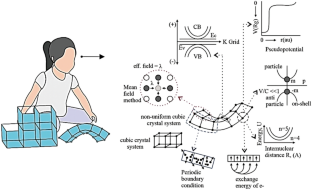
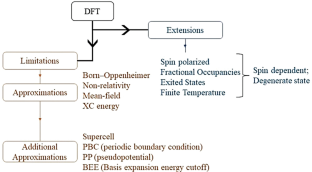
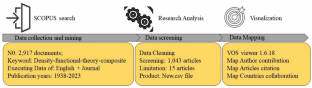
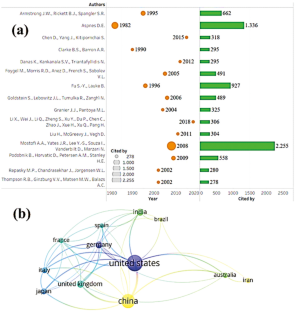
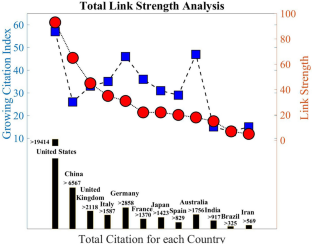
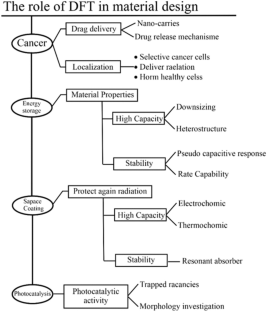
Similar content being viewed by others
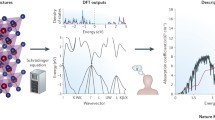
Computational predictions of energy materials using density functional theory
Article 11 January 2016
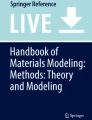
The Materials Project: Accelerating Materials Design Through Theory-Driven Data and Tools
Chapter © 2018
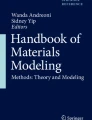
The Materials Project: Accelerating Materials Design Through Theory-Driven Data and Tools
Chapter © 2020
Data Availability
All data and materials generated or analyzed during this study are included in this article.
References
- P. Hohenberg and W. Kohn, Phys. Rev. 136, B864 (1964). Google Scholar
- W. Kohn and L.J. Sham, Phys. Rev. 140, A1133 (1965). Google Scholar
- K. Hanasaki, Z.A. Ali, M. Choi, M. Del Ben, and B.M. Wong, J. Comput. Chem. 44, 980–987 (2022). Google Scholar
- W. Jamshed, M.R. Eid, A.F. Al-Hossainy, Z. Raizah, E.S.M. Tag El Din, and T. Sajid, Sci. Rep. 12, 18130 (2022). Google Scholar
- M.D. Ganji, Sh. Mirzaei, and Z. Dalirandeh, Sci. Rep. 7, 4669 (2017). Google Scholar
- E. Nemati-Kande, A. Pourasadi, F. Aghababaei, S. Baranipour, A. Mehdizadeh, and J.J. Sardroodi, Sci. Rep. 12, 19972 (2022). Google Scholar
- Y. Kang, Z. Mao, Y. Wang, C. Pan, M. Ou, H. Zhang, W. Zeng, and X. Ji, Nat. Commun. 13, 2425 (2022). Google Scholar
- B. Geng, J. Hu, Y. Li, S. Feng, D. Pan, L. Feng, and L. Shen, Nat. Commun. 13, 5735 (2022). Google Scholar
- S.V. Gupta, V.V. Kulkarni, and Md. Ahmaruzzaman, Sci. Rep. 13, 3009 (2023). Google Scholar
- N. Kitchamsetti, M.S. Ramteke, S.R. Rondiya, S.R. Mulani, M.S. Patil, R.W. Cross, N.Y. Dzade, and R.S. Devan, J. Alloys Compd. 855, 157337 (2021). Google Scholar
- Z. Gan, J. Yin, X. Xu, Y. Cheng, and T. Yu, ACS Nano 16, 5131 (2022). Google Scholar
- Z. Gan, L. Xia, J. Yin, Y. Gao, X. Feng, G. Meng, Y. Cheng, and X. Xu, ACS Appl Energy Mater 5, 15452 (2022). Google Scholar
- L. Pernigoni, U. Lafont, and A.M. Grande, CEAS Space Journal 13, 341 (2021). Google Scholar
- M.-I. Trappe and R.A. Chisholm, Nat. Commun. 14, 1089 (2023). Google Scholar
- L.H. Thomas, Math. Proc. Cambridge Philos. Soc. 23, 542 (1927). Google Scholar
- A. Ardiansyah, D. Tahir, H. Heryanto, B. Armynah, H. Salah, A. Sulieman, and D.A. Bradley, Radiat. Phys. Chem. 207, 110835 (2023). Google Scholar
- V. Seymour, Front. Public Health 4, 260 (2016). Google Scholar
- A. Chaves, J.G. Azadani, H. Alsalman, D.R. da Costa, R. Frisenda, A.J. Chaves, S.H. Song, Y.D. Kim, D. He, J. Zhou, A. Castellanos-Gomez, F.M. Peeters, Z. Liu, C.L. Hinkle, S.-H. Oh, P.D. Ye, S.J. Koester, Y.H. Lee, Ph. Avouris, X. Wang, and T. Low, NPJ 2D Mater. Appl. 4, 29 (2020). Google Scholar
- S. Luo, T. Li, X. Wang, M. Faizan, and L. Zhang, WIREs Comput. Mol. Sci. 11, e1543 (2021). Google Scholar
- Y. Li and K. Yang, Energy Environ. Sci. 12, 2233 (2019). Google Scholar
- Q.M. Dowling, H.E. Volkman, E.E. Gray, S. Ovchinnikov, S. Cambier, A.K. Bera, B. Sankaran, M.R. Johnson, M.J. Bick, A. Kang, D.B. Stetson, and N.P. King, Nat. Struct. Mol. Biol. 30, 72 (2023). Google Scholar
- A.H.-W. Yeh, C. Norn, Y. Kipnis, D. Tischer, S.J. Pellock, D. Evans, P. Ma, G.R. Lee, J.Z. Zhang, I. Anishchenko, B. Coventry, L. Cao, J. Dauparas, S. Halabiya, M. DeWitt, L. Carter, K.N. Houk, and D. Baker, Nature 614, 774 (2023). Google Scholar
- P. Giannozzi, S. Baroni, N. Bonini, M. Calandra, R. Car, C. Cavazzoni, D. Ceresoli, G.L. Chiarotti, M. Cococcioni, I. Dabo, A. Dal Corso, S. de Gironcoli, S. Fabris, G. Fratesi, R. Gebauer, U. Gerstmann, C. Gougoussis, A. Kokalj, M. Lazzeri, L. Martin-Samos, N. Marzari, F. Mauri, R. Mazzarello, S. Paolini, A. Pasquarello, L. Paulatto, C. Sbraccia, S. Scandolo, G. Sclauzero, A.P. Seitsonen, A. Smogunov, P. Umari, and R.M. Wentzcovitch, J. Phys. Condens. Matter 21, 395502 (2009). Google Scholar
- W. Yi, G. Tang, X. Chen, B. Yang, and X. Liu, Comput. Phys. Commun. 257, 107535 (2020). MathSciNetGoogle Scholar
- S.P. Ong, W.D. Richards, A. Jain, G. Hautier, M. Kocher, S. Cholia, D. Gunter, V.L. Chevrier, K.A. Persson, and G. Ceder, Comput. Mater. Sci. 68, 314 (2013). Google Scholar
- I.G. Ryabinkin, E. Ospadov, and V.N. Staroverov, J. Chem. Phys. 147, 164117 (2017). Google Scholar
- B. Kanungo, P.M. Zimmerman, and V. Gavini, Nat. Commun. 10, 4497 (2019). Google Scholar
- M.K. Harbola and V. Sahni, J. Chem. Educ. 70, 920 (1993). Google Scholar
- P. Singh and M.K. Harbola, Oxford Open Mater. Sci. 1, itab018 (2020). Google Scholar
- V.P. Gupta, Principles and Applications of Quantum Chemistry (Academic Press, Boston, 2016), p155. Google Scholar
- M. Tayyab, A. Hussain, Q. Ula-Asif, and W. Adil, Comput. Condens Matt. 23, 00469 (2020). Google Scholar
- H.-Y. Huang, M. Broughton, J. Cotler, S. Chen, J. Li, M. Mohseni, H. Neven, R. Babbush, R. Kueng, J. Preskill, and J.R. McClean, Science 376, 1182 (2022). MathSciNetGoogle Scholar
- M. Soniat, D.M. Rogers, and S.B. Rempe, J. Chem. Theory Comput. 11, 2958 (2015). Google Scholar
- P. Borlido, J. Schmidt, A.W. Huran, F. Tran, M.A.L. Marques, and S. Botti, NPJ Comput. Mater. 6, 96 (2020). Google Scholar
- M.E. Tuckerman, P.J. Ungar, T. von Rosenvinge, and M.L. Klein, J. Phys. Chem. 100, 12878 (1996). Google Scholar
- M. Boero, A. Bouzid, S. Le Roux, B. Ozdamar, and C. Massobrio, Molecular Dynamics Simulations of Disordered Materials (Springer, Switzerland, 2015), p33. Google Scholar
- C. Lorenz and N.L. Doltsinis, Handbook of Computational Chemistry (Springer, Netherlands, 2012), p195. Google Scholar
- P. Gori-Giorgi, M. Seidl, and G. Vignale, Phys. Rev. Lett. 103, 166402 (2009). Google Scholar
- D. Gibney, J.-N. Boyn, and D.A. Mazziotti, J. Phys. Chem. Lett. 13, 1382 (2022). Google Scholar
- J. Wang and E.J. Baerends, Phys. Rev. Lett. 128, 013001 (2022). Google Scholar
- H.J. Silverstein, A. Huq, M. Lee, E.S. Choi, H. Zhou, and C.R. Wiebe, J. Solid State Chem. 221, 216 (2015). Google Scholar
- T. Balcerzak, and K. Szałowski, J. Magn. Magn. Mater. 513, 167157 (2020). Google Scholar
- K. Okhotnikov, T. Charpentier, and S. Cadars, J. Cheminform. 8, 17 (2016). Google Scholar
- S.Y. Docherty, W.D. Nicholls, M.K. Borg, D.A. Lockerby, and J.M. Reese, Proc. Inst. Mech. Eng. C J. Mech. Eng. Sci. 228, 186 (2014). Google Scholar
- P.L. Barclay and D.Z. Zhang, J. Comput. Phys. 435, 110238 (2021). Google Scholar
- P. Schwerdtfeger, theoretical Chemistry and Physics of Heavy and Super heavy Elements (Springer, Dordrecht, 2003), p399. Google Scholar
- K.F. Garrity, J.W. Bennett, K.M. Rabe, and D. Vanderbilt, Comput. Mater. Sci. 81, 446 (2014). Google Scholar
- P. Kraus, J. Chem. Theory Comput. 16, 5712 (2020). Google Scholar
- Liang and M. Head-Gordon, J. Phys. Chem. A 108, 3206 (2004). Google Scholar
- A. Förster and L. Visscher, J. Comput. Chem. 41, 1660 (2020). Google Scholar
- A. D. Becke, in (2014), pp. 175–186.
- C.A. Ullrich and Z. Yang, Braz. J. Phys. 44, 154 (2014). Google Scholar
- C. Adamo and D. Jacquemin, Chem. Soc. Rev. 42, 845 (2013). Google Scholar
- T. Yoshikawa, T. Doi, and H. Nakai, J. Chem. Phys. 152, 244111 (2020). Google Scholar
- M. Teplitskiy, E. Duede, M. Menietti, and K.R. Lakhani, Res. Policy 51, 104484 (2022). Google Scholar
- E. Fermi, Zeitschrift Fr. Physik 48, 73 (1928). Google Scholar
- A.A. Mostofi, J.R. Yates, Y.-S. Lee, I. Souza, D. Vanderbilt, and N. Marzari, Comput. Phys. Commun. 178, 685 (2008). Google Scholar
- D.E. Aspnes, Thin Solid Films 89, 249 (1982). Google Scholar
- S. Fu, Compos. Sci. Technol. 56, 1179 (1996). Google Scholar
- M. Foygel, R.D. Morris, D. Anez, S. French, and V.L. Sobolev, Phys. Rev. B 71, 104201 (2005). Google Scholar
- N. Manikandan, V.P. Suresh Kumar, S. Siva Murugan, G. Rathis, K. Vishnu Saran, and T.K. Shabariganesh, Mater. Today Proc. 47, 4682 (2021). Google Scholar
- U.A. Bukar, M.S. Sayeed, S.F.A. Razak, S. Yogarayan, O.A. Amodu, and R.A.R. Mahmood, MethodsX 11, 102339 (2023). Google Scholar
- J. Leeming, Nature 556, 139 (2018). Google Scholar
- E.R. Tsai, A.N. Tintu, D. Demirtas, R.J. Boucherie, R. de Jonge, and Y.B. de Rijke, Crit. Rev. Clin. Lab. Sci. 56, 458 (2019). Google Scholar
- K. Dai, S. Shen, and C. Cheng, Sci. Rep. 12, 3644 (2022). Google Scholar
- J.A. Hubbell and R. Langer, Nat. Mater. 12, 963 (2013). Google Scholar
- A.M. Ferrari, C. Pisani, F. Cinquini, L. Giordano, and G. Pacchioni, J. Chem. Phys. 127, 174711 (2007). Google Scholar
- Y.S. Meng and M.E. Arroyo-de-Dompablo, Energy Environ. Sci. 2, 589 (2009). Google Scholar
- P. Sengupta and I. Manna, Trans. Indian Inst. Met. 72, 2043 (2019). Google Scholar
- S. Razzaghi, M. Vafaee, B. Kharazian, and M. Nasrollahpour, Sci. Rep. 13, 3323 (2023). Google Scholar
- Y. Zhou, X. Jia, D. Pang, S. Jiang, M. Zhu, G. Lu, Y. Tian, C. Wang, D. Chao, and G. Wallace, Nat. Commun. 14, 297 (2023). Google Scholar
- S. Fleischmann, J.B. Mitchell, R. Wang, C. Zhan, D. Jiang, V. Presser, and V. Augustyn, Chem. Rev. 120, 6738 (2020). Google Scholar
- Z. Zhu, T. Jiang, M. Ali, Y. Meng, Y. Jin, Y. Cui, and W. Chen, Chem. Rev. 122, 16610 (2022). Google Scholar
- H. Demiryont and D. Moorehead, Sol. Energy Mater. Sol. Cells 93, 2075 (2009). Google Scholar
- K. Bašnec, L.S. Perše, B. Šumiga, M. Huskić, A. Meden, A. Hladnik, B.B. Podgornik, and M.K. Gunde, Sci. Rep. 8, 5511 (2018). Google Scholar
- H. Kim, K. Cheung, R.C.Y. Auyeung, D.E. Wilson, K.M. Charipar, A. Piqué, and N.A. Charipar, Sci. Rep. 9, 11329 (2019). Google Scholar
Acknowledgements
We thank all the researchers focusing on the field of material design. Everything you discover makes the world a better place. There is no research funding for this work.
Author information
Authors and Affiliations
- Department of Physics, Hasanuddin University, Makassar, 90245, Indonesia Heryanto Heryanto, Ardiansyah Ardiansyah, Roni Rahmat & Dahlang Tahir
- Heryanto Heryanto